Vaporization is a fundamental process in various thermal systems, and understanding the mechanisms behind it is crucial in the design of efficient heat exchange systems. One such phenomenon, nucleate boiling, plays a critical role in the heat transfer process when vaporization occurs directly at the heating surface. This article will explore the concept of nucleate boiling, its characteristics, the mechanisms that drive it, its applications in heat transfer systems, and the factors that affect its performance.
1. Introduction to Vaporization and Boiling
Boiling is a phase transition from the liquid phase to the vapor phase. It occurs when a liquid is heated to its boiling point, and vapor bubbles form within the liquid and rise to the surface. There are two main types of boiling:
Nucleate Boiling: This is the process where vaporization occurs directly at discrete sites on the heated surface. It is characterized by the formation of small vapor bubbles at nucleation sites, which then grow and detach.
Film Boiling: This occurs when a continuous vapor layer forms between the liquid and the heating surface, preventing direct contact between the two. In film boiling, heat transfer efficiency is lower because of the insulating effect of the vapor layer.
Nucleate boiling is particularly important in high-performance heat exchange systems due to its efficient heat transfer capabilities. The transition from nucleate boiling to film boiling can dramatically affect the efficiency of thermal systems, which is why understanding the dynamics of nucleate boiling is essential.
2. Mechanisms of Nucleate Boiling
In nucleate boiling, vaporization takes place directly at the heating surface, typically on small cavities, microscopic pits, or other imperfections on the surface, known as nucleation sites. When the temperature of the heating surface exceeds the liquid’s boiling point, the liquid in contact with the surface starts to vaporize. This process occurs in several steps:
2.1 Nucleation Site Formation
Nucleation sites are small cavities or imperfections on the surface of the heating material. These sites trap tiny vapor bubbles when the temperature is high enough for vaporization to occur. For nucleate boiling to occur effectively, these sites must be in contact with the liquid. They serve as locations where the liquid can form vapor bubbles more easily.
2.2 Bubble Formation and Growth
As the liquid touches the heated surface, the liquid closest to the surface heats up and vaporizes into bubbles. The bubbles form at the nucleation sites and grow as they absorb more heat from the surface. These growing bubbles accumulate in the liquid, causing an increase in local vapor volume.
The size of the bubbles increases as the liquid beneath the bubble reaches its boiling point. The heat transfer is efficient because the vapor bubbles formed in nucleate boiling carry away thermal energy from the surface as they move toward the surface of the liquid.
2.3 Bubble Detachment and Rise
Once the bubbles reach a certain size, they detach from the surface and rise through the liquid. As they move upward, they displace the surrounding liquid, which leads to mixing and enhanced heat transfer. This process of bubble formation, growth, and detachment helps in transporting heat away from the heating surface.
The continual process of bubble formation and detachment creates a convective heat transfer mechanism. It significantly enhances the efficiency of heat exchange, as the moving bubbles help to stir the liquid, making the heat transfer more uniform and increasing the rate at which heat is carried away from the surface.
3. Characteristics of Nucleate Boiling
Nucleate boiling is characterized by several key features that make it a highly efficient heat transfer process:
3.1 High Heat Transfer Coefficient
One of the main advantages of nucleate boiling is its high heat transfer coefficient. This means that heat is transferred from the surface to the liquid much more efficiently than in other modes of boiling. This is because the vapor bubbles formed at the heating surface create turbulence and enhance liquid circulation, which improves the overall heat transfer.
In nucleate boiling, the heat flux increases significantly with the temperature difference between the surface and the liquid. The formation of vapor bubbles at nucleation sites results in a much higher thermal conductivity compared to other boiling regimes.
3.2 Localized Vaporization
Unlike film boiling, where a vapor layer insulates the heating surface, nucleate boiling occurs at localized sites directly on the surface. This means that the heat transfer is not hindered by a continuous vapor barrier, and the liquid comes into direct contact with the heated surface. The formation of multiple vapor bubbles at different nucleation sites spreads the heat transfer evenly across the surface.
3.3 Critical Heat Flux
As the heating surface continues to increase in temperature, the heat flux (the rate at which heat is transferred) also increases. However, there is a critical heat flux beyond which nucleate boiling transitions to film boiling. At this point, the entire surface becomes covered with a vapor layer, reducing the effectiveness of heat transfer.
The critical heat flux represents the maximum heat transfer rate that can be achieved with nucleate boiling. Exceeding this flux can result in a dramatic reduction in heat transfer efficiency and can cause the heating surface to overheat, potentially leading to thermal damage.
3.4 Temperature Gradients
The temperature gradient in nucleate boiling is significant. The liquid near the surface is heated to its boiling point, while the rest of the liquid remains cooler. As vapor bubbles form and detach, they carry away heat, creating a dynamic temperature gradient within the liquid. This gradient drives the convective currents, further enhancing the heat transfer process.
4. Applications of Nucleate Boiling
Nucleate boiling plays a crucial role in many applications, particularly in systems requiring efficient heat transfer. Some common applications of nucleate boiling include:
4.1 Cooling Systems
Nucleate boiling is often used in cooling systems for electronic devices, nuclear reactors, and industrial machinery. The efficient heat removal from surfaces through nucleate boiling helps in preventing overheating and ensuring optimal performance. In applications like power plants, heat exchangers, and cooling towers, nucleate boiling is employed to remove large amounts of heat from hot surfaces.
4.2 Thermal Management in Electronics
In the world of electronics, miniaturization and increased power densities require advanced cooling techniques. Nucleate boiling is used in thermal management systems to dissipate heat away from components like microprocessors, which generate significant amounts of heat during operation. These cooling systems often utilize small-scale boiling processes to maintain the safe operating temperature of components, preventing damage due to heat.
4.3 Heat Exchangers
Heat exchangers rely on nucleate boiling to transfer heat between two fluids without allowing them to mix. In these systems, a fluid is heated and passed through a surface, where it vaporizes. The vaporized fluid then transfers heat to a second fluid passing through the heat exchanger, where it condenses and releases the heat. The nucleate boiling process is particularly useful in two-phase heat exchangers, where both liquid and vapor phases are involved.
4.4 Power Generation
In power generation, nucleate boiling is essential in the operation of steam boilers and turbines. Water is heated to generate steam, and nucleate boiling helps in the efficient generation of steam by allowing rapid vaporization of water. This steam is then used to drive turbines that generate electricity. The efficiency of the boiling process directly impacts the overall efficiency of the power plant.
5. Factors Affecting Nucleate Boiling Performance
Several factors can influence the efficiency and performance of nucleate boiling. These include:
5.1 Surface Characteristics
The surface roughness and material properties of the heating surface play a crucial role in nucleate boiling. Surfaces with small pores, cavities, or roughness tend to have a higher number of nucleation sites, leading to more efficient boiling. Smooth surfaces, on the other hand, can inhibit bubble formation and reduce the overall heat transfer rate.
5.2 Liquid Properties
The properties of the liquid, such as its thermal conductivity, viscosity, and surface tension, also affect nucleate boiling. Liquids with low surface tension promote the formation of bubbles, while liquids with higher viscosity may reduce bubble movement and reduce heat transfer efficiency.
5.3 Heat Flux and Temperature
As the heat flux increases, so does the rate of bubble formation. However, exceeding the critical heat flux can lead to a transition from nucleate boiling to film boiling, which decreases heat transfer efficiency. The temperature of the heating surface must be carefully controlled to ensure the system operates within the nucleate boiling regime.
5.4 Subcooling
Subcooling refers to the degree to which the liquid temperature is below its boiling point. Higher subcooling generally increases the intensity of nucleate boiling, as the liquid has more potential to absorb heat and undergo vaporization. However, excessive subcooling can reduce bubble formation and the overall heat transfer rate.
6. Conclusion
Nucleate boiling is a highly efficient heat transfer process that occurs directly at the heating surface, where vaporization takes place at discrete nucleation sites. This process is crucial in various applications, from power generation and electronics cooling to industrial heat exchangers. Its efficiency in transferring heat makes it indispensable in systems requiring rapid heat removal.
By understanding the mechanisms, characteristics, and influencing factors of nucleate boiling, engineers and designers can optimize heat exchange systems to improve their performance and prevent issues like surface overheating. As thermal management requirements continue to evolve, nucleate boiling will remain a cornerstone of high-efficiency heat transfer technologies.
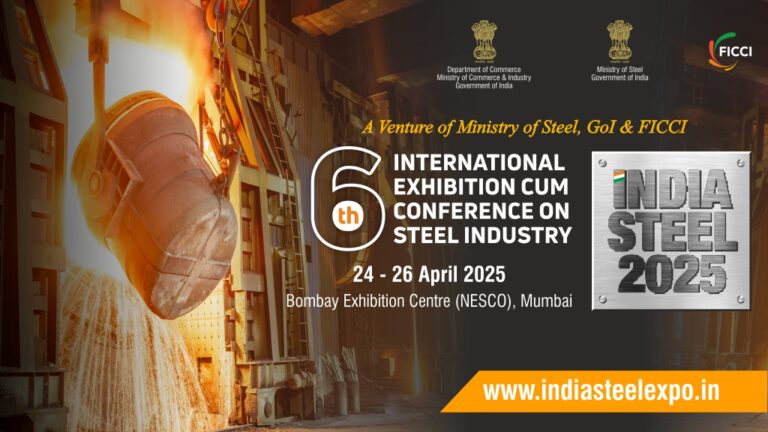